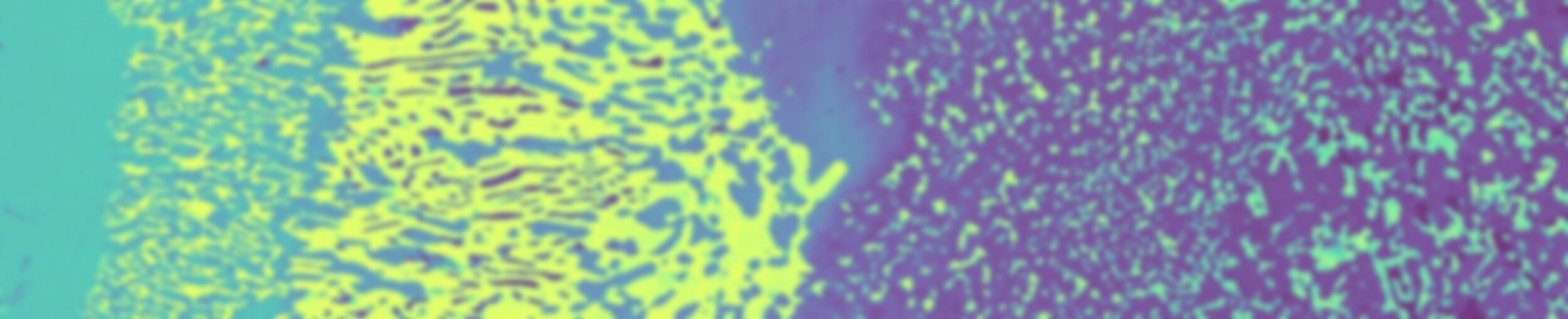
.1.
Refractory Rocks in Gneiss Domes
Background
The bulk of continents is composed of quartzofeldspathic material (granites, gneisses, migmatites) that typically only record the last stages of its geologic history, as the minerals that make them up readily transform as the rock re-equilibrate to low-pressure, high-temperatures in the late stages of a continental crust-altering event. Some rock types are chemically more refractory to change- the minerals that they are made up of do not break down and recrystallize as easily when exposed to high-temperature. These types of rocks, such as mafic (iron- and magnesium-rich rocks) or aluminous rocks, have greater potential to preserve a more complete, earlier part of their history compared to the quartzofeldspathic rocks. In the field, they are often associated, but clearly retain a different memory of their history. The goal of my research is to use these chemically refractory rocks to investigate earlier and potentially more complete records of continental crust transformation, critical in understanding the ‘full picture’ of the geodynamic and tectonic processes by which they transform.
Why do we care?
Continents are the substrate of life- for us humans, as well as other organisms before us and those to come. Our planet is the only one that we know of to have continents. Why that is remains an unanswered scientific question at large.
Mountain building events - or orogenesis - are one of the Earth’s ways of reusing or recycling what it is already made of. As continents collide, the rocks composing the outermost layer of the planet deform and their mineralogical makeup undergoes transformations during metamorphism as conditions of pressure (P) or depth, and temperature (T) or heat change. As rock reach high temperatures, they melt and migrate vertically from deep to shallow levels of the crust, moving large volumes of material and heat from depth to the surface. However, how far material flows horizontally at depth, possibly bringing hot and deep rocks from several hundreds of kilometers away from their ultimate place of rest and crystallization, is not well understood.
Better understanding the trajectory and magnitude of this earlier, lateral migration of material at depth has fundamental implication for our understanding of how large mountain-forming events like the one involved in forming the Himalayas redistribute rocks within the crust, bettering our understanding of why and how continents persist over geologic time.
Research Questions
-
1 | Do refractory rocks retain a comprehensive history of their transformation during mountain-building events?
-
2 | What is the temporal and structural relationships between associated mafic and felsic rocks?
-
3 | What was the trajectory and magnitude of material transport laterally at depth prior to exhumation?
Methods
In order to answer these questions, I use a variety of approaches, tools, and analytical techniques to investigate rocks at the field (km to m), sample (cm to mm), and micro (individual minerals and distinct domains within them) scales. I use:
Field-mapping and microscopy to look at field-scale trends in rock textures and structures;
In-situ laser-ablation split-stream inductively coupled plasma mass spectroscopy (LASS-ICP-MS) petrochronology to investigate the timing and micro-chemical environments in which datable minerals such as zircon and rutile likely formed during metamorphism;
Secondary Ion Mass Spectroscopy (SIMS) to analyze oxygen-isotopes of minerals such as zircon and garnet to determine what the fluids present during their formation can tell us about the setting of their formation;
Electron probe microanalysis (EPMA) to investigate the micro-chemical compositions and variations of minerals present in the rocks, which tell us about the P and T conditions under which they formed
A combination of scanning electron microscopy (SEM) and electron backscatter diffraction (EBSD) to investigate the strength and preferred orientation of minerals in rocks to understand how they deformed, coupled with anisotropy of magnetic susceptibility (AMS), a method to quantify rock fabrics using the magnetic properties of minerals that makeup the rocks.
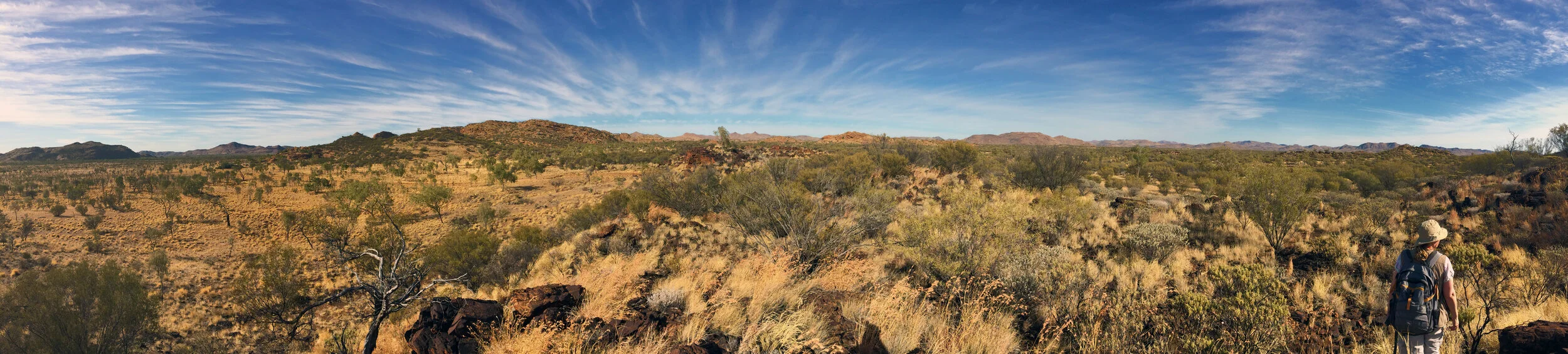
Domes Under Investigation
Entia Dome, central Australia
The Entia Dome is an exceptionally well exposed, nearly equant gneiss dome that contains mafic rocks that record a range of pressures (P). This exceptional preserved record of rocks from different depths (lithostatic P) provides a framework to investigate how rocks deform during exhumation within the 3-D architecture of a domal structure such as the Entia.
I study the magnetic fabrics of mafic rocks recording variable P, combined with SEM-EBSD analyses to investigate the crystallogrpahic preferred orientation (CPO) of mafic minerals to reconstruct the trajectory and intensity of deformation throughout the stages of exhumation of rocks in the Entia Dome.
The primary goal of this project is to develop a method to reliably relate magnetic fabrics in amphibole-bearing rocks to the intensity and strain geometry captured at the time of their crystallization at variable depths. This will provide a better understanding of the overall process of dome emplacement, vertical recycling of material during dome formation, as well as a method to investigate deformation in amphibole-bearing rocks in other field localities with potentially wide applications.
Montagne Noire dome, France
The Montagne Noire contains eclogites, mafic rocks that form exclusively at high-pressure (>50 km deep) in the crust and are relics of the deep origins of dome-forming material. Along with key textural information, I use a high-resolution, multi-methods, multi-systems approach to study the timing of protolith formation and metamorphism (zircon, rutile in-situ LASS-ICP-MS) paired with fluid history recorded by individual zircon domains (O-isotopes analyses of garnet in-situ and zircon in grain-mount) to determine the source/origin of these eclogites and their relative trajectory during deep crustal flow to eclogite facies, prior to exhumation in the dome, at the southernmost margin of the Variscan orogen foreland during the late stages of orogeny.
I also use quantitative EPMA mapping to investigate the vertical trajectory of eclogites on the exhumation path by using the chemical composition of minerals in symplectites, fine intergrowths of minerals that formed as the result of the breakdown of a precursor phase, in this case the high-P mineral omphacite destabilized during pressure-decrease. Using this quantitative data with the textural context of the mineral associations at the micron-scale, we calculate P and T conditions recorded by the rocks along the exhumation path, and investigate the differences in textures and mineral assemblages preserved in symplectites in eclogites from different parts of the dome.